
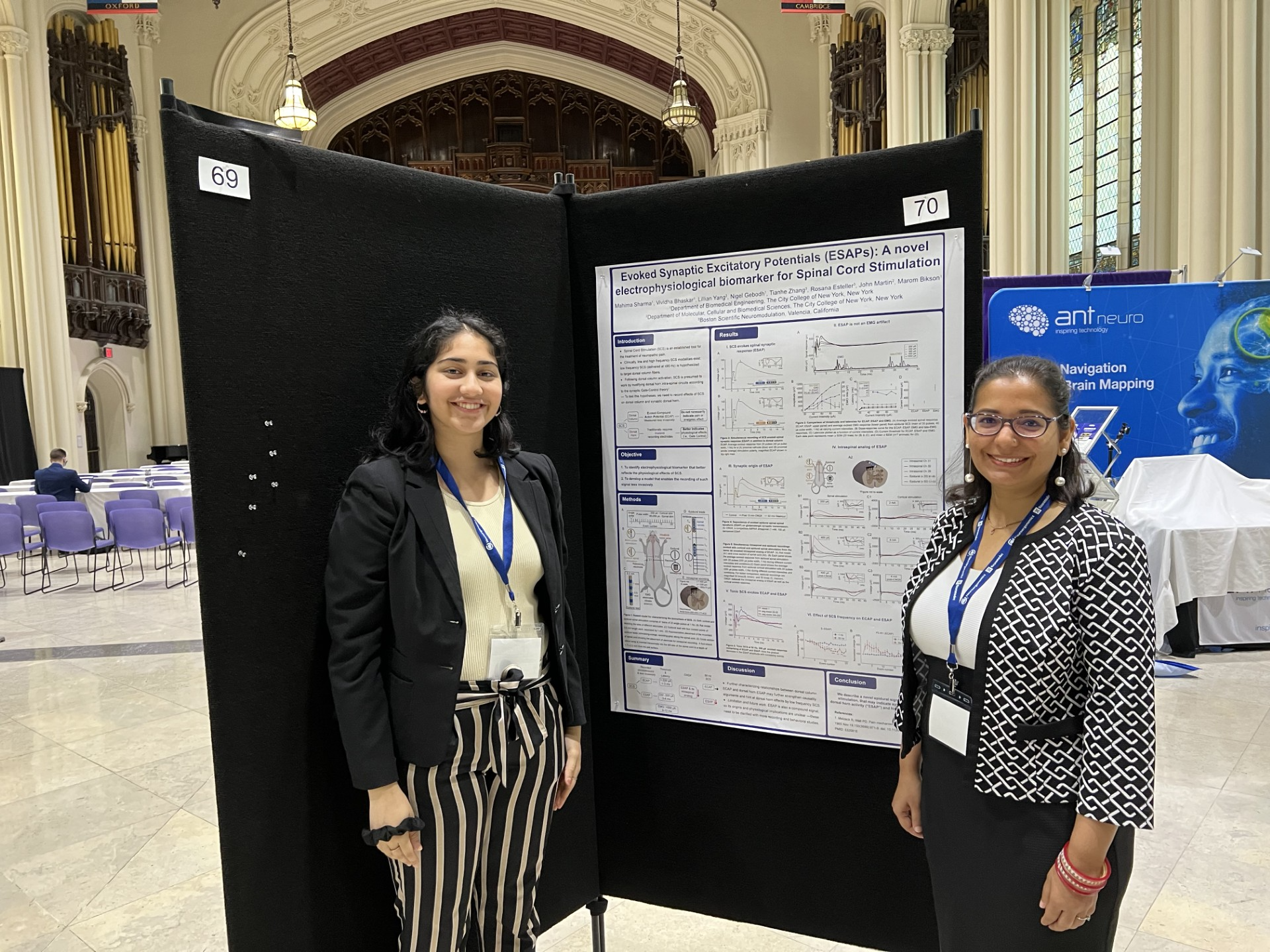
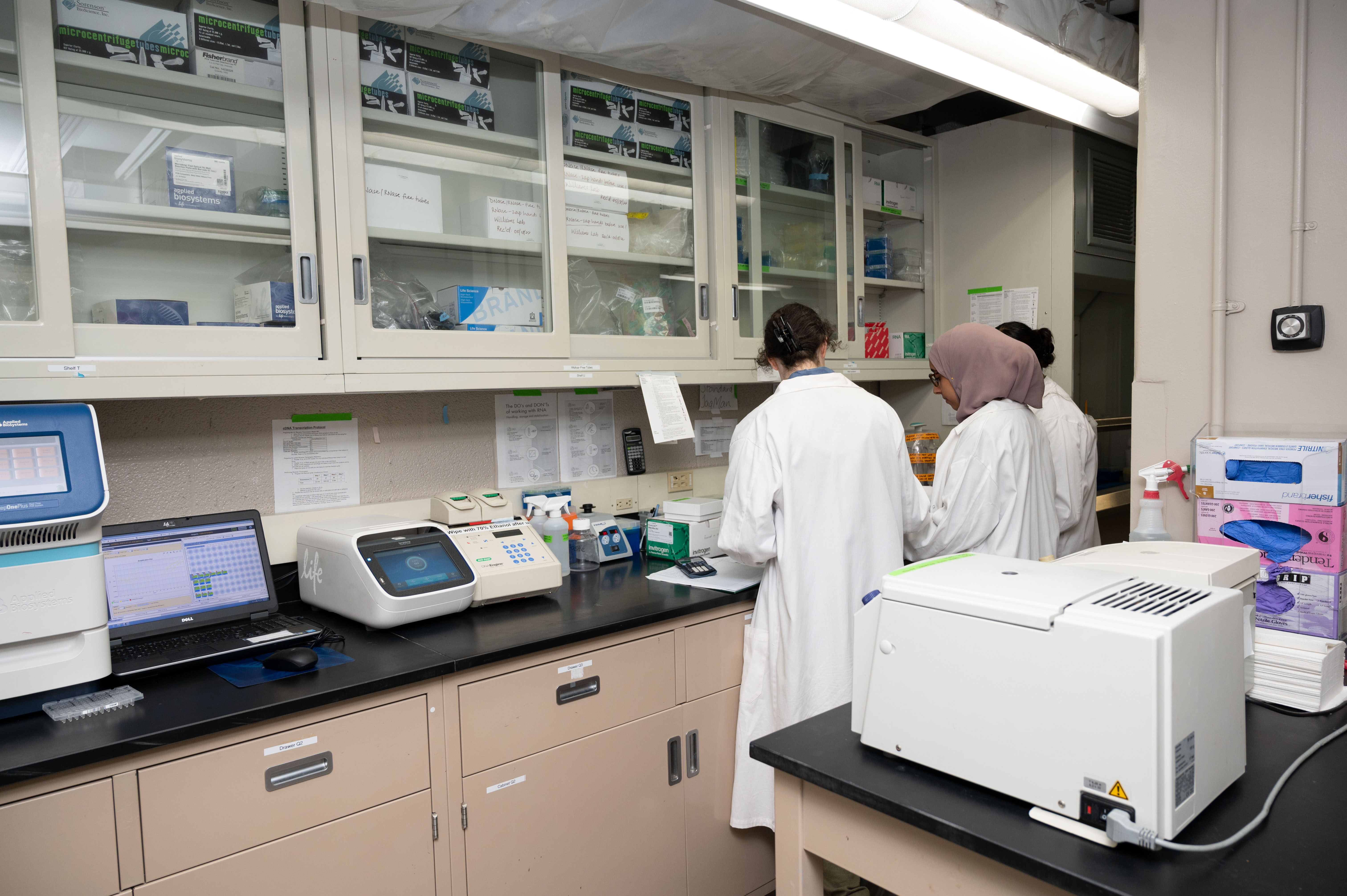
BME @ CCNY is incredibly research active with each of our faculty receiving federal funding from agencies such as the NIH, NSF, and DoD. In 2023, the department held almost $8 million in research grants, which is ~$670,000 per research-active faculty member. This complements the ~180 publications and 9 pending or issued patents from our faculty. Our faculty have an average of 9,900 citations each.
Cardiovascular Engineering
Cardiovascular Engineering is motivated by the desire to understand the mechanisms of the cardiovascular system, particularly with respect to cardiovascular disease and microcirculation. Interests include cellular mechanotransduction, transport phenomenon, and vessel biomechanics.
-
Multiscale Biomechanics and Functional Imaging Laboratory (Dr. Luis Cardoso)
The Laboratory of Multiscale Biomechanics and Functional Imaging aims to integrate biomechanics, bioinstrumentation, signal and image processing to study health disorders in the osteoarticular and cardiovascular fields. Our laboratory is involved in developing experimental, theoretical and numerical multiscale approaches to determine the biomechanical and functional competence of living tissues before and after their degeneration occurs (i.e., bone fragility, osteoarthritis and rupture of thin caps on atherosclerotic blood vessels). To integrate these interdisciplinary goals, our laboratory is equipped with a new Phased Array Ultrasound System, electronics and machine shop, computational infrastructure for three-dimensional imaging processing and Finite Element Modeling, and a wet lab for basic histology processing. Furthermore we are developing an Acoustic Microscope and a small animal facility that will include an operating room, anesthesia machine and a PC-controlled Continue Passive Motion device.
-
Microcirculation Laboratory (Dr. Bingmei Fu)
In the microcirculation laboratory we perform in vivo permeability measurements on intact single microvessels to investigate the mechanisms of microvessel permeability related diseases such as tumor metastasis, thrombosis, strokes, brain injuries and Alzheimer’s disease. We use cutting-edge fluorescence image techniques such as in vivo intracellular calcium concentration imaging to explore signal transduction events. We use quantitative fluorescence video, confocal and photometer microscopy to measure microvessel permeability and cell migration rate change caused by mechanical, physical and chemical stimuli. Information obtained from the experiments serves to develop and test mathematical models of microvascular transport based on fundamental principles of biomechanics, in order to advance basic understanding of the role of the microcirculation in maintaining life. The analysis forms the basis to understand various diseases from a cellular and molecular point of view. It also provides information to design new drugs and drug delivery methods.
-
Cardiovascular Dynamics and Biomolecular Transport Laboratory (Professor Emeritus Dr. John Tarbell)
The Wallace Coulter Laboratory for Cardiovascular Dynamics and Biomolecular Transport studies the role of fluid mechanics and transport processes in the physiological and pathophysiological functions of the cardiovascular system. One of our major efforts is to understand the influence of fluid dynamics in the initiation and progression of atherosclerosis, a degenerative disease of the large human arteries which leads to heart attacks and strokes. We are investigating the fluid mechanics of arteries and the response of arterial cells (endothelial and smooth muscle cells) to fluid mechanical forces using cell culture models in vitro and computer simulations. We were the first group to compute the fluid flow shear stresses on smooth muscle cells (SMC’s) induced by transmural flow and have subsequently exposed cultured SMC’s to similar stress environments in defined flow fields to determine their biomolecular responses. In complementary research, we have pioneered in vitro studies of convection and diffusion of macromolecules across monolayers of endothelial cells which form the blood contacting surface of all blood vessels. We were the first group to clearly demonstrate that the transport properties of the endothelial layer are very sensitive to their fluid mechanical environment and will respond to changes in fluid shear stress. Studies of the biomolecular mechanisms underlying these responses are in progress.
-
Cardiovascular Transport (Professor Emeritus Dr. Sheldon Weinbaum)
Dr. Weinbaum's primary approach to problems is through mathematical modeling and the application of principles of fluid and solid mechanics and mass transport, but always in close association with experimentalists and real data. He has made major contributions in developing the “leaky junction” theory of transendothelial transport and has pioneered a new understanding of the classical “Starling Law”. The latest work from the Weinbaum group addresses the critical problem of “vulnerable plaque” rupture in the coronary arteries, and has been done in collaboration with Dr. Luis Cardoso.
Musculoskeletal Biomechanics
Musculoskeletal Biomechanics research focuses on bone tissue and orthopaedic biomechanics. Interests include bone and skeletal mechanical loading states, mechanosensory systems, fluid flow, imaging and microarchitecture.
-
Tissue Mechanics Laboratory (Dr. Susannah Fritton)
The focus of the Tissue Mechanics Laboratory is to understand the adaptive response of bone to altered physiological conditions. The lab uses a combined experimental and computational approach to investigate interstitial fluid flow around osteocytes, which is believed to play a role in the mechanism by which bone tissue detects external mechanical stimuli and deposits or resorbs bone as needed. A major thrust of the current research effort is to understand how bone mechanotransduction is altered in postmenopausal and disuse osteoporosis. An ongoing project in collaboration with investigators at the Hospital for Special Surgery involves relating bone microstructure and cellular-level changes to alterations of interstitial fluid flow in osteoporotic bone. Another current project investigates whether reduced mechanical loading causes changes in the bone microporosities and osteocyte environment that diminish bone’s ability to detect mechanical loading. Techniques developed in the lab are also being applied to the field of cancer drug delivery in a project with collaborators at Memorial Sloan-Kettering Cancer Center that investigates the efficacy of applying mechanical loading to enhance delivery of therapeutic agents to bone tumors.
Lab website: http://sfritton-lab.ccny.cuny.edu
-
Multiscale Biomechanics and Mechanobiology Laboratory (Dr. Alessandra Carriero)
The research focus of this laboratory is to provide a comprehensive understanding of mechanics and adaptation of biological tissues and organs, such as bone, joints and ears, in healthy, aging and disease so to aid in the development of new treatment strategies for health disease and disorders. For the purpose we use a multidisciplinary and multiscale approach involving experimental and computational mechanical engineering methods, material science approaches, high-resolution imaging, clinical data and biological analyses to reveal how abnormalities in the multiscale hierarchy of biological tissues can cause whole organ failure, and how mechanical forces influence tissue growth, shape and function. Our research aims to inform clinicians on ways to correct or prevent failure and abnormalities in biological tissues, such as bone fragility and deformities, before they become debilitating.
-
Bone and Joint Laboratory (Dr. Mitchell Schaffler)
The major emphasis of the Bone and Joint Laboratory is to understand how skeletal tissues (bone, ligament, cartilage) develop, maintain and repair themselves in order to meet mechanical demands throughout life. We focus on the cellular processes that control characteristic architectural features of these tissues, and how they respond to physical and metabolic challenges in aging and in diseases such as osteoporosis, genetic defects and diabetes. We are currently investigating fatigue and repair in bone and tendon, with specific emphasis on discovering how living cells in these tissues detect and repair wear and tear damage before it accumulates to the point of tissue failure. We are also examining how osteocytes (the tissue-resident bone cells) influence bone’s mechanical function, both directly by controlling local matrix composition, and indirectly by governing local bone remodeling activity. In related studies, we are examining how osteocytes function as mechanosensors that allow bone to perceive and react to mechanical stimuli. Experimental approaches used in the Bone and Joint Laboratory focus on both the cell and tissue levels, and include mechanical loading studies, finite element and mathematical modeling, microscopy and other imaging modalities, cell culture and molecular biology.
Nanotechnology & Biomaterials
Nanotechnology and Biomaterials research ranges from the development of microfluidic devices and tissue engineered constructs to smart biomaterials and targeted drug delivery techniques for clinical application. Additionally, these systems are being used to understand cellular behavior with respect to differentiation, migration, and macroscopic cell-tissue interactions.
-
Connective Tissue Engineering Laboratory (Dr. Steve Nicoll)
Research in the Connective Tissue Engineering Laboratory incorporates the principles of cell and molecular biology, materials science, and mechanical engineering toward the development of living tissue surrogates for connective tissue restoration. A prevailing theme in each of the major research thrusts is understanding how environmental stimuli (i.e., mechanical forces and biochemical mediators) direct the differentiation of novel progenitor cells (i.e., human dermal fibroblasts, fetal cells) toward specialized lineages, including cartilage and bone cells. Efforts are also focused on the design of new biomaterials, such as photo-cross linked cellulosic hydrogels, to modulate cellular phenotype and functional tissue growth.
-
Laboratory of Microfluidic HTS Technology and Tissue Engineering (Dr. Sihong Wang)
One focus of this laboratory is directed at the development of microfluidic cell arrays to study sigaling pathways, such as apoptosis and inflammation, for the high throughput screening (HTS) of drugs using current discoveries in biomedical sciences and advanced technologies in BioMEMS. The long term collaboration with Memorial Sloan-Kettering Cancer Institute facilitates the development of microfluidic 3D cell arrays for HTS. Another focus of the lab is to investigate thermal effects on 3D tissue regeneration in synthetic extracellular matrices using stem cells and explore the role of heat shock proteins in the tissue development, injury protection and repair. Bone marrow mesenchymal stem cell isolation and characterization are routinely performed in the lab. Currently we are focused on bone and cartilage regeneration.
-
Immune Nanomedicine Laboratory (Dr. Ryan M. Williams)
The Immune Nanomedicine Laboratory designs, characterizes, and deploys novel nanotechnologies to treat and diagnose diseases. Specifically, the lab focuses on disrupting and detecting immune and inflammatory processes in kidney disease and cancer. One focus of the lab is to develop kidney-targeted polymeric nanoparticles as therapeutic tools for renal diseases. Another area of concentration is developing ex vivo and implantable optical nanosensor devices using single-walled carbon nanotubes (SWCNT) as diagnostic tools for cancers and related diseases. Each of these areas of concentration combine rational material design, using materials as tools to understand biological processes in disease, and translational aspects including efficacy, pharmacology, and safety.
Lab website: https://williamslab.ccny.cuny.edu
Neural Engineering and Imaging
Neural Engineering applies engineering principles to fundamental questions in neuroscience and to the development of treatments for neurological disorders. Specific interests include analyzing nervous system function at multiple levels including single channel, single cell, tissue, whole animal, and human cognitive levels. We integrate experimental insights with computational modeling and pursue translational research through the NYCBE.
Our group leverages the tremendous rapid prototyping and fabrication infrastructure of the CCNY Grove School of Engineering and the translational expertise of NYCBE hospitals.
Addressing medical needs and developing innovative medical device treatments requires a rational pipeline that spans basic scientific discovery, IP, medical device design, regulatory, and clinical trials. The CCNY Neural Engineering group and our partners are one of the few academic centers in the world with integrated expertise spanning these areas.
-
Applied Bioelectricity, Neurophysiology, and Medical Devices Laboratory (Dr. Marom Bikson)
Neural engineering includes the application of engineering principles to solve fundamental problems in neuroscience and to produce practical solutions to human neurological problems. The aims of this laboratory include: 1) establishing the mechanisms by which weak (e.g. power line, mobile phone) and strong (electrical prosthesis, deep brain stimulation) electric fields modulate brain function; 2) elucidating the neuronal network dynamics, including non-synaptic mechanisms, facilitating emergent physiological (“gamma”/cognition) and pathological (epilepsy) network oscillations; 3) rapid-prototyping and validation of innovative therapeutic and diagnostic technologies. The Neurophysiology laboratory is equipped with state-of-the-art electrophysiology/microscopy equipment which allows the monitoring of bioelectrical activity generated by populations of neurons and by single visualized neurons. The lab also employs shared resources of the Neural Engineering Group (Bikson, Parra, Kelly) for whole-brain electrophysiology (EEG), eye tracking, electrical circuit design and high-end computing resources.
Lab website: https://neuralengr.org -
Signals and Computation Laboratory (Dr. Lucas Parra)
Current experimental techniques focus on interpreting and modulating brain activity in humans non-invasively using electro-encephalography and trans-cranial electrical stimulation, or in short, "reading" and "writing" the brain with electric fields. The work is often coupled with auditory and visual psychophysics and always incorporates computational or mathematical models, or in short, "modeling" the brain. To establish basic cellular mechanism it also relies on in-vitro electrophysiology. For the work with human subjects the laboratory has a sound-damped electromagnetically shielded room, a portable 128-channel system and eye tracker with drivers for real-time analysis and closed-loop stimulation and adaptive displays. Research grade audio equipment is available to perform auditory perception experiments. Our custom equipment for trans-cranial electric stimulation (AC and DC) with simultaneous EEG recording is unique inthe world. In addition to data collection the laboratory performs data analysis of brain signals and images as well as computational modeling of spiking networks using a variety of computational tools. The lab leverages shared resources of the Neural Engineering Group (Bikson, Parra, Dmochowski) for electrophysiology on the single-neuron, population and whole-brain levels, microscopy, eye tracking, electrical circuit design and high-end computing resources with modeling software.
Lab website: https://parralab.org -
Laboratory of Dr. Jacek Dmochowski
Dr. Dmochowski's research develops novel techniques for (i) non-invasively stimulating the brain and (ii) decoding neural signals. We are testing the transcranial application of ultrasound and near-infrared lasers to restore or enhance physiological activity in the central nervous system. Our efforts in neural decoding adopt a machine learning approach to infer brain states from neuroimaging data collected in naturalistic settings. We make extensive use of biophysical modeling of the human head as well as multivariate statistical techniques to optimize interventions and increase the sensitivity of our decoding methods. The research is expected to translate to new treatments for psychiatric and neurological disorders.
Lab website: https://dmochow.github.io/
Last Updated: 08/30/2024 09:56